DYNAMIQS
OVERVIEW
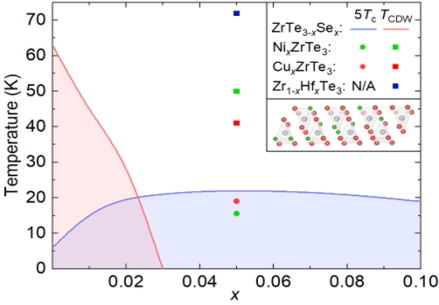
Phase diagram of TCDW and 5Tc as a function of doping (Se and Hf) and intercalation (Ni, Cu). The data was adopted from Ref. [7, 8, 9]. Inset: 1D- chain in crystal structure of ZrTe3-xSex.
The uniqueness of TMTs is rising from the peculiarity of their crystal structure. The basic structural units of these materials are 1D chains aligned parallel to crystallographic b-axis, formed from MX3 prisms interconnected with shearing M – X bonds, where M stands for transition metal and X stands for chalcogen element. The way the chains form and group together is a parameter that enables the classification of TMTs and determines their behavior and properties. The anisotropic symmetry of TMTs is especially convenient for strain application along direction of 1D-chains and direction orthogonal to it. In that way it is possible to induce the formation of new structures in preferred direction, and improve desired properties, such as magnetism, CDW or SC state [1, 2, 3]. So far it was proposed that the electron-phonon interaction is the principal mechanism behind the formation of CDW state [4].
Specifically in the material of interest, ZrTe3 , the CDW transition occurs at 63 K [5]. Untypically, the SC transition is filamentary to the bulk, i.e., at first (around 4 K), quasi-1D superconductivity forms as a consequence of pairing along a-axis, and by decreasing the temperature down to 2 K the bulk superconductivity is established [6].
By applying hydrostatic pressure on ZrTe3 , the TCDW abruptly decreases below 2.5 K at 5 GPa. Initially, the Tc is suppressed with increasing pressure, but above 5 GPa the SC order is reestablished. Furthermore, when temperature exceeds the room temperature, a weak low-dimensional magnetism attributed to the structural defects and/or edge-states still can be observed in ZrTe3 [7, 8, 9]. The substitution of Te with the Se atoms causes the chemical pressure which as a result leads to the suppression of CDW phase in ZrTe3-xSex . With increasing Se content x an increase in Tc is observed, reaching its maximum of 4.4 K for x=0.04, the so-called quantum critical point (QCP), where CDW vanishes completely [10, 11]. This research suggests superconductivity mediated by charge fluctuations. Furthermore, intercalation of ZrTe3 with Ni and Cu also leads to CDW suppression and almost doubling of the Tc . The CDW gap characteristic for the CDW transitions, experiences significant energy shift in Ni-intercalated compounds [9, 12, 13]. The isostructural compound to ZrTe3 , HfTe3 undergoes a CDW transition at much higher temperature (⁓ 93K) and SC is not observed down to 50 mK [14]. By doping ZrTe3 with Hf atoms the TCDW increases significantly, for approximately 10 K and SC phase is suppressed.
One of the techniques that offers insights into lattice, charge, and spin dynamics, as well as their interplay in materials with strong electron correlations, is the inelastic scattering of visible light, commonly known as Raman scattering [15, 16]. Each excitation is governed by selection rules that limit its observation to specifically determined scattering channels. By manipulating the scattering geometry (the polarization of incident and scattered light, as well as the sample orientation) it is possible to resolve and assign excitations. By integrating the uniaxial strain field as an additional external parameter, we can obtain new information that can lead to a better understanding of these systems.
[1] J. Cenker et al., Reversible strain-induced magnetic phase transition in a van der Waals magnet, Nat. Nanotechnol. 17, 256–261 (2022).
[2] S. Gao et al., Atomic-scale strain manipulation of a charge density wave, PNAS 115 (27), 6986-6990 (2018).
[3] M. Mito et al., Large enhancement of superconducting transition temperature in single-element superconducting rhenium by shear strain, Sci Rep 6, 36337 (2016).
[4] Y. Hu, et al., Charge density waves and phonon-electron coupling in ZrTe3, Phys Rev B. 91(14):144502 (2015).
[5] S. Takahashi et al., Transport and elastic anomalies in ZrTe3, Solid State Commun. 49(11):1031–1033 (1984).
[6] K. Yamayaet al., Mixed bulk-filament nature in superconductivity of the charge-density-wave conductor ZrTe3, Phys Rev B. 85(18):184513 (2012).
[7] R. Yomoet al., Pressure effect on competition between charge density wave and superconductivity in ZrTe3: Appearance of pressure-induced reentrant superconductivity, Phys. Rev. B 71, 132508 (2005).
[8] S. L. Gleasonet al., Structural contributions to the pressure-tuned charge-density-wave to superconductor transition in ZrTe3: Raman scattering studies, Phys. Rev. B 91, 155124 (2015).
[9] X. Yuet al., Fast and controlled growth of two-dimensional layered ZrTe3 nanoribbons by chemical vapor deposition, CrystEngComm, 21, 5586-5594 (2019).
[10] S. Cuiet al., Multiband nodeless superconductivity near the charge-density-wave quantum critical point in ZrTe3−xSex, Chinese Phys. B 25 077403 (2016).
[11] X. Zhu et al., Superconductivity and Charge Density Wave in ZrTe3−xSex, Sci Rep 6, 26974 (2016).
[12] X. Lei et al., Raising Tc in charge density wave superconductor ZrTe3 by Ni intercalation, Europhysics Letters, 95(1), p.17011 (2011).
[13] C. Mirri et al., Excitation spectrum in Ni- and Cu-doped ZrTe3, Phys. Rev. B 89, 035144 (2014).
[14] Y. Liu et al., Thermal transport and mixed valence in ZrTe3 doped with Hf and Se. Appl Phys Lett. 120(2):022601 (2022).
[15] D. P. Devereaux and R. Hackl, Inelastic light scattering from correlated electrons, Rev. Mod. Phys. 79, 175 (2006)
[16] N. Lazarević and R. Hackl, Fluctuations and pairing in Fe-based superconductors: light scattering experiments, J. Phys.: Condens. Matter 32, 413001 (2020).